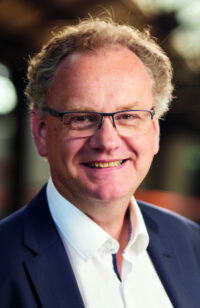
While questions remain over the efficacy and safety of lithium-ion batteries in stationary applications, professor Thomas Maschmeyer, founder and principal technology advisor at Gelion Technologies, looks at how zinc-bromide redox can meet the energy tri-vector of sustainability, cost, and performance capability in the stationary energy storage industry.
The climate crisis is front and centre of plans to accelerate the transition to a low-carbon economy, with many governments legislating for net-zero emissions by mid-century. To achieve this transition to carbon-neutrality, storage of renewable energy will need to be adopted everywhere: supporting industry and domestic power usage, the emergence of green hydrogen, stabilising the grid and efficiently managing renewable energy demand. How to effect this change will be exercising the minds of leaders as they review the Glasgow Agreement presented at the United Nations Climate Change Conference COP26 last October/November.
Lithium-ion batteries have become the technology of choice in powering much of the stationary energy storage market until now, especially at scale. Despite its undoubted success, the nature of lithium-ion chemistry continues to give rise to concerns about thermal runaway and fires— as well as the need to maintain tight charging and discharging windows, while closely regulating the battery’s temperature.
This means overall system costs are affected by the need to take these limitations into account in terms of capital and operating expenditure. The fact that they need significant resources to manufacture— and are hard to recycle at the end of their life— presents further environmental issues down the line. Ever-increasing supply pressure from the EV market adds to the complexity.
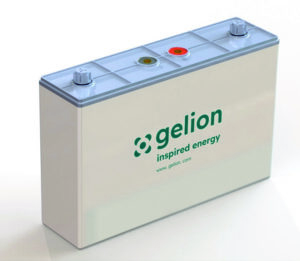
Non-flow zinc-bromide battery technology offers a solution to these issues for stationary energy storage.
Zinc-bromide redox chemistry uses materials that are benign, cost-efficient and available globally from non-conflict zones. Previous iterations of zinc-bromide redox batteries relied on complex and expensive support technologies to maintain the system’s flow chemistry. However, new proprietary non-flow gel battery chemistries first described 150 years ago, has turned the technology into a modern and efficient energy storage system, without the need for cumbersome external support systems. This battery technology has an excellent levelised cost of energy storage with characteristics that are particularly well suited to solar energy shifting and a real potential to challenge the incumbent energy storage systems in these markets.
Clean, dependable power
Many technologies will be needed to achieve net-zero targets; batteries are a key technology, offering solutions to many of the integration, operation and supply problems, allowing for flexible market-driven approaches to achieve decarbonisation.
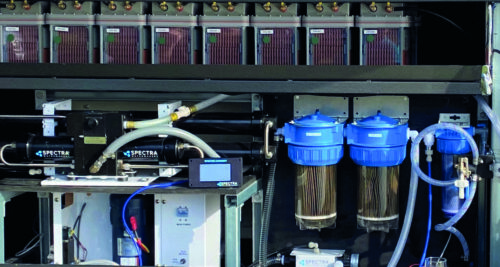
The rising demand for batteries has most visibly been driven by the expansion in electric vehicle production for the consumer market. Even so, battery storage and its role in integrating clean energy into the energy mix is also beginning to take off— for example as coal-fired power stations are not replaced or are even taken off the grid before the end of their design life.
However, to meet the demand for the transition to renewables in the electrification of transport and heavy industry, battery production will need to be increased at a global scale.
Rystad Energy estimates new global battery manufacturing capacity will need to increase to 20 times the current production capacity— or more than 20 terawatt-hours by 2050— broadly divided by transport and energy storage. The research institution reports that 78% of global CO2 emissions could be partly addressed by energy storage.
Lithium-ion technology continues to drive the expanding energy storage markets— both stationary and mobile. However, alternative battery chemistries, such as zinc bromide, offer highly compelling opportunities in the stationary energy storage market due to their inherent advantages.
Gelion’s zinc-bromide technology enables a non-flow battery format and uses a lead-acid type architecture, design and set of manufacturing principles, allowing for simple and low-cost integration into existing manufacturing capacity. This will enable rapid global roll-out via manufacturing partnerships. This conceptual approach brings substantial benefits to cost, scaling and operability.
The commercial technology is similar to lead-acid in style, constructed as a 12V multi-cell battery with a capacity up to 1.2kWh, an RTE (round-trip efficiency) of about 85-90%, and can operate from temperatures well below freezing all the way up to 50°C, without the need for expensive cooling systems. The cells can be combined to service applications from tens of kWhs to hundreds of MWhs in low-, medium- and high-voltage scenarios. Data from the US Energy Information Agency in 2019 shows that this RTE is higher than the average monthly rate of the US utility-scale battery fleet (82%) and pumped-hydro storage facilities (79%).
Zinc-bromide batteries can be repeatedly discharged from 100% charge to zero volts without harming performance, displaying a high ‘abuse tolerance’ in terms of state-of-charge management.
This attribute also greatly improves electrical safety, as transport, installation and maintenance can all be performed with the battery carrying no electrical potential. Additionally, this enables the battery to chemically ‘reset’ during a full discharge, resulting in reduced or no capacity fade and maintenance requirements. This is especially attractive for remote locations. Such ‘conditioning’ may take place at monthly intervals or longer, despite daily cycling. Some zinc-bromide batteries can also be stored at full discharge for extended periods, remaining fully functional and available for recharge to resume normal operations.
Having this attribute makes zinc bromide especially useful in hard to decarbonise sectors, such as mining and heavy industry, or in harsh, remote off-grid environments that make it difficult for companies to shift from diesel-fuelled generators. It presents a new opportunity for these industries to reduce greenhouse gas emissions associated with some of their site activities and begin the process of reducing their overall footprint.
Energy storage for the planet
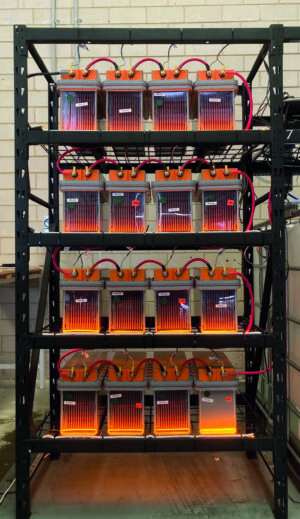
When the zinc-bromide battery’s electrolyte is fully discharged, it forms a benign aqueous salt, posing minimal risk to the environment. When charged, the battery contains zinc and an inherently stabilised form of bromine.
Bromine, which is commonly used in fire retardant materials, is one of the core components of Gelion’s non-flow gel battery. When in use, the battery’s electrolyte itself is flame retardant. Despite the bromine generated during charging being highly reactive (giving the battery its power), it is safely encapsulated within a molecular sponge, while remaining accessible to the energy storage process.
Once discharged, the electrolyte can be handled as easily as any other concentrated salty solution, enabling cells to be disassembled for the recycling of just about all its various components— similar to lead-acid batteries, but without the issues around lead toxicity and sulfuric acid management.
Non-flow gel batteries will be among the safest to operate and simplest to recycle once reaching end-of-life, while also maintaining lifetime carbon efficiency throughout its usage. These factors, inherent to the technology, align closely with corporate environmental, social, and governance (ESG) outcomes, and match with six of the UN Sustainable Development Goals in delivering an environmentally friendly solution that meets energy-storage needs for today and tomorrow.
The production of zinc-bromide batteries emits low greenhouse gas (GHG) emissions per kWh of stored and cycled energy throughout its life cycle. The GHG emissions produced during the manufacturing process are 0.029 kg CO2 emissions (CO2e) per kWh cycled during the life of the battery, or 9.9 kg CO2e per kilogram of battery. When averaged out, the energy storage system has a GHG footprint 61% lower than lithium-ion batteries and 85% lower than lead-acid batteries. The materials that make up the batteries primarily drive the savings made by this technology when compared to other technologies.
Overall, the unique advantages in terms of low environmental impact (materials, manufacturing and recycling), high safety, broad operability parameters and inherent robustness, give rise to a commercially and environmentally compelling storage solution that can service many of the urgent demands in the current and rapidly emerging global stationary energy storage markets.
Technology underpins the transition

If we are to achieve the transition to net-zero, successfully decarbonise the economy and overcome the burgeoning climate crisis, we need a diverse energy mix that is built upon technological innovation. Energy storage— particularly in the stationary market— is a technology that will underpin much of this transition, transforming the way renewable energy is deployed and managed at grid level.
Furthermore, many solutions are available now and should be implemented quickly to ensure that decarbonisation moves at a pace that aligns with the urgent need to meet global climate goals. The zinc-bromide design developed by Gelion enables low production costs and reduces the time to market by leveraging the established manufacturing ecosystems of lead-acid batteries. This means that such enabling stationary storage technology does not need to be invented; it is here today and poised for global scale-up.
As world governments consider how the global route to net-zero is mapped out following negotiations at last year’s COP26 conference in Glasgow and agree upon measures for tackling greenhouse gas emissions, energy storage should be seen as a keystone technology that can unleash the true potential of clean power and ensure that affordable, scalable solutions are available to all.
As companies bring viable, low-cost and commercial solutions to the table, public and private stakeholders should move now to adopt these technologies to make the transition to a clean energy mix and meet legally binding net-zero targets.
Professor Maschmeyer’s biography
Thomas Maschmeyer was awarded the Australian Prime Minister’s Prize for Innovation (2020) – the Australian Academy of Science’s David Craig Medal (2021) and the Australian Financial Review Sustainability Prize (2021).
He was also named as one of the top-10 international People of the Year: who mattered in higher education in 2020 by Times Higher Education and ranks as #15 in the world’s Top Influential Chemists 2010-2020.
He concurrently holds the position of professor of chemistry at the University of Sydney, where he established and leads the Laboratory of Advanced Catalysis for Sustainability and served as founding director of the University of Sydney Nano Institute (2015-2018).
In 2011, he was elected youngest Foreign Member of the Academia Europe as well as Fellow of the Australian Academy of Sciences, the Australian Academy of Technological Sciences and Engineering, the Royal Australian Chemical Institute (RACI) and, in 2014, of the Royal Society of NSW.